Dynamic response of HMX under shock loading
The thermomechanical response of the energetic material under impact is closely related to its safety and reliability during processing, storage, and transportation. The goal of this project is to establish a multiscale and multiphysics model to predict the shock loading resistance of the energetic material. In our previous work, we developed a pressure-dependent anisotropic hyperelasticity and crystal plasticity model to incorporate pressure sensitivity while predicting plastic dissipation. It is found that the external pressure has a strong influence on the plastic dissipation under shock loading, which cannot be captured by the traditional crystal plasticity model. The material point method (MPM) is a hybrid Eulerian-Langrangian method, which is immune to mesh distortion and hence suitable for large deformation simulation. Also, MPM provides an automatic no-penetration no-slip contact algorithm which enables the pore-collapse simulation. In our preliminary results with the Johnson-Cook model, it is found that the heat-induced strain softening and the shear band can be reproduced with MPM. We are currently working on introducing the anisotropic phase-field fracture model into the MPM to simulate the fracture near the collapsing pores and related heat generation.
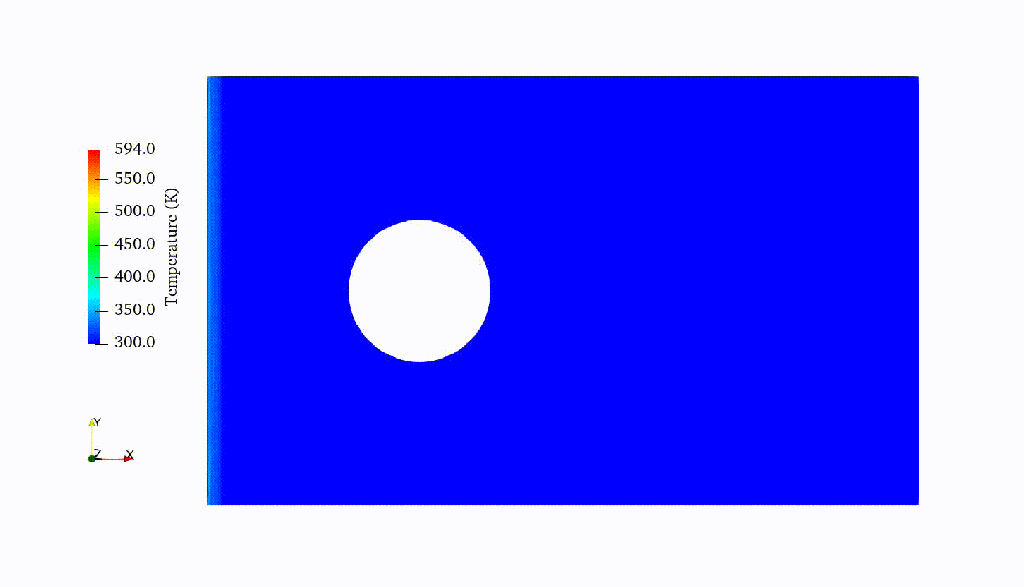
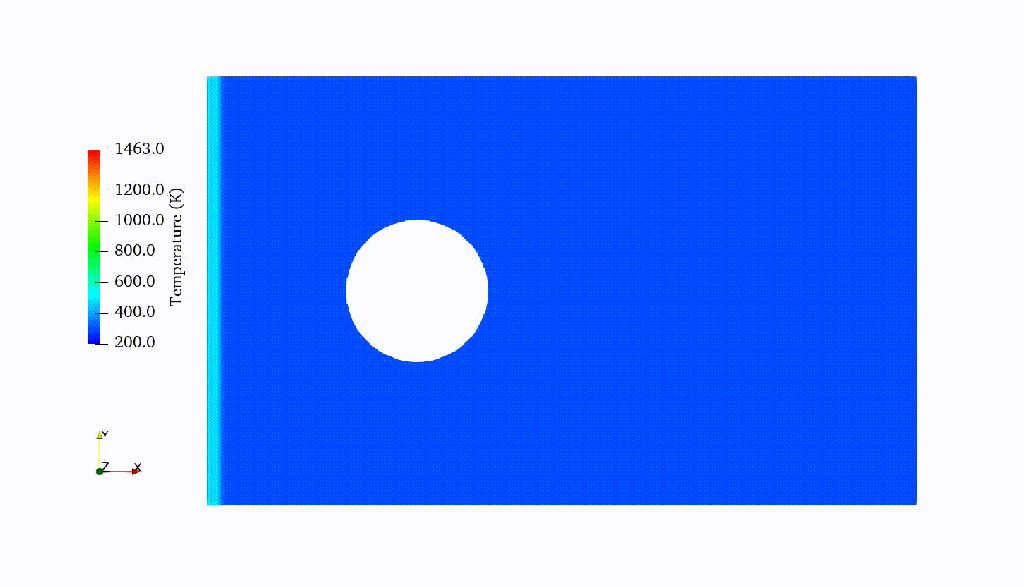
Long-term creep prediction in rock salt
The thermo-hydro-mechanical behavior of rock salt is strongly dependent on the microstructural attributes and micro-mechanics of the halite crystal that forms the rock salt. The desired engineering properties, such as the high thermal conductivity and the low permeability, and self-healing of rock salt are both attributed to the crystalline nature. In a geological system composed of salt or other crystalline rocks, individual grains or crystals are the fundamental building blocks that form polycrystal assembles. The deformation of these assemblies is then related to both the deformation of each individual crystal and the grain boundary motion. As a result, thermo-mechanical responses at the microscopic scale, such as intergranular and intragranular fractures, as well as plastic slips, dislocation, and the creeping due to precipitation may have significant implications for large scale engineering applications. While there is a multitude of macroscopic phenomenological models attempted to capture the complicated responses of rock salt, a multiscale approach that rightfully treated rock salt as polycrystalline materials may allow more robust predictions on the macroscopic anisotropic responses of rock salt in the finite deformation range. The goal of this work is to derive, formulate, predict, verify and validate a polycrystalline model for rock salt and propose a multiscale method to bridge the spatial and temporal scales.


Microtextured region in Ti-6242
The titanium alloy Ti-6242 (Ti-6Al-2Sn-4Zr-2Mo) has been the structural material of choice for use in high-pressure compressors for gas turbine engines of aircraft due to its high strength-to-weight ratio and excellent high-temperature mechanical properties. However, Ti-6242 is susceptible to dwell fatigue at low temperature due to crack growth on low-angle boundaries along the primary α grains, in particular small faceted cracks that link up to form a “quasi-cleavage” surface. These low-angle boundaries occur within so-called microtextured regions (MTR) that consist of many neighboring primary α grains with similarly oriented c-axes of the hexagonal close-packed atomic lattice. Post-mortem observations of specimens have revealed facet clusters along the fracture surface within the MTR. In this work, the crystal plasticity finite element method in WARP3D is used to model the processing of MTR within Ti-6242. Several configurations of MTR within representative microstructures have been simulated under single and multiple loading axes to determine the critical strain to break down the MTR and introduce more disorientation into the microstructure. These and ongoing computational studies provide a complement to experimental data from AFRL and mill processors that demonstrate a load direction dependence on the texture evolution of this material.
